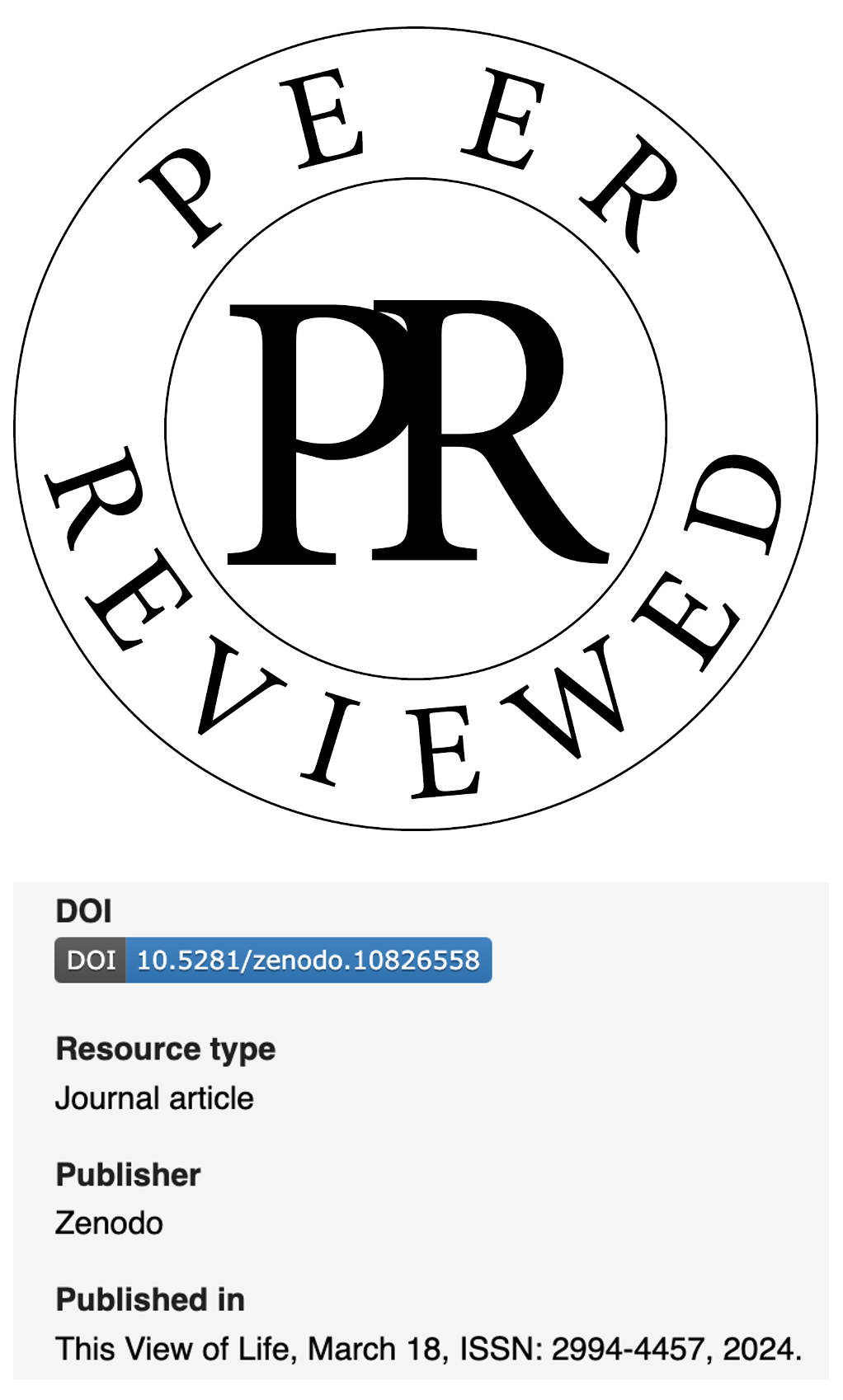
Abstract
Nearly every individual and society seeks to work toward positive change, but these efforts often perversely result in dysfunctional outcomes, especially when different aspirations collide with each other. This is also true in nature, where adaptations that evolve by natural selection do not necessarily result in well-functioning animal societies and multispecies communities. A classic book on this subject is Adaptation and Natural Selection, published by George C. Williams in 1966. Williams forcefully asserted that adaptation at any level of a multitier hierarchy of units (e.g., individuals, single species social groups, or multispecies ecosystems) requires a process of selection at the same level and tends to be undermined by selection at lower levels. This can be called Williams’ Rule. While Williams’ Rule is widely appreciated by evolutionary biologists, it is little known in other topic domains, such as the human-related academic disciplines and pragmatic efforts to accomplish positive change. The purpose of this article is to make Williams’ Rule more widely known, which can improve any positive change effort, no matter what topic domain or scale.
Introduction
It is part of human nature to seek positive change for ourselves, either informally in our everyday lives or collectively through our institutions and formulations of public policy. Yet, these well-meaning efforts often perversely result in dysfunctional outcomes. Like the characters of so many folk tales, we end up regretting what we wished for, based on unforeseen consequences. Pathological outcomes are especially likely when individuals and groups attempt to seek advantage at the expense of others.
These problems are not restricted to humans. In all species, natural selection results in individual organisms that are exquisitely adapted to survive and reproduce in their environments. Yet, their individual-level adaptations do not necessarily combine to produce adaptive single-species societies or multi-species communities. It’s not as if adaptations above the level of the individual never evolve. Instead, special conditions are required.
Decades were required for evolutionary biologists to think clearly about this issue. The Christian worldview that preceded Darwin assumed that, as God’s creation, nature is harmoniously organized from top to bottom, from the tiniest creatures to the stars in heaven.
After Darwin, many biologists continued to assume that nature is adaptively organized above the level of the individual organism, such as single-species social groups, entire species, and multi-species ecosystems. The fact that special conditions are required for higher-level adaptations to evolve was not widely appreciated1 until George C. Williams published his book Adaptation and Natural Selection in 1966, which became a classic within the field of evolutionary biology (see Sober and Wilson 2011 and Agren 2021 for modern appraisals).
Williams reminded his readers that natural selection is based on relative fitness. It doesn’t matter how well an individual survives and reproduces in absolute terms; only that it does so better than other individuals in its vicinity. Adaptations that confer a relative fitness advantage among individuals within a social group are frequently not good for the group, species, or ecosystem. The paradigmatic example is the comparison between altruistic behaviors, which benefit others at the expense of oneself, and selfish behaviors, which benefit oneself at the expense of others. Natural selection within groups favors selfishness, not altruism.
Williams acknowledged that altruism and other “for the good of the group” behaviors can evolve, but only by a process of between-group selection. He expressed his conviction in no uncertain terms:
"It is universally conceded by those who have seriously concerned themselves with this problem that such group-related adaptations must be attributed to the natural selection of alternative groups of individuals and that the natural selection of alternative alleles within populations will be opposed to this development. I am in entire agreement with the reasoning behind this conclusion. Only by a theory of between-group selection could we achieve a scientific explanation of group-related adaptations." (p. 91-92)
A statement this definitive deserves to be called a law or a rule. I will call it Williams’ Rule to admit the possibility of the occasional exception.
Williams’ Rule: Group-level adaptations require a process of group-level selection.
What Williams asserted for two-level selection can be expanded to a multi-tier hierarchy of groups within groups within groups. Frame-shifting downward, selection can take place among genes or cell lineages within organisms (e.g., cancers), in addition to between organisms. Frame-shifting upward, selection can take place among whole multispecies ecosystems, such as microbiomes. The multilevel version of Williams’ Rule is:
Adaptation at any level of a nested hierarchy of units requires a process of selection at that level and tends to be undermined by selection at lower levels.
The history of what followed the publication of Adaptation and Natural Selection is complex, as I will outline in more detail below. The bottom line, I will argue, is that Williams’ Rule has withstood the test of time. It is possible for supra-individual biological and human social systems to evolve into adaptive units, but only by a process of system-level selection that is sufficiently strong to counteract disruptive selection within the system.2
While Williams’ Rule is widely known and largely accepted among evolutionary biologists who study nonhuman species and multispecies communities, it is little-known in the human-related academic disciplines and myriad attempts to accomplish positive change in real-world settings. Yet, Williams’ Rule is just as relevant to the genetic and cultural evolution of our species as it is to the rest of life. Hence, the purpose of this article is to provide a summary and update of Adaptation and Natural Selection for a general audience. If I succeed, then you, my reader, will have an improved ability to identify both the presence and absence of functional organization at multiple scales and contexts, and to evolve cooperative systems that do not currently exist. While this article is largely a tutorial, I will also touch upon a number of important issues that need to be resolved at the expert level.
A Brief History of Williams’ Rule in Evolutionary Biology
The history of Williams’ rule in evolutionary biology is complex because Williams made two strong claims. The first is what I have dubbed Williams’ Rule: the theoretical claim that higher-level adaptations require higher-level selection and tend to be undermined by lower-level selection. The second was the empirical claim that higher-level selection is almost invariably weak, compared to lower-level selection. The second claim led Williams to argue that while altruism and other “for the good of the group” traits can evolve in principle, they seldom evolve in actuality.
For a period of decades following the publication of Adaptation and Natural Selection, most evolutionary biologists were persuaded that both claims were true, so that most adaptations in nature must be explained as varieties of individual self-interest (Sober and Wilson 1998, Okasha 2006; Borello 2010). This became known as the theory of individual selection. I was one of a handful who argued in favor of group selection as a significant evolutionary force, which makes it ironic that I am now assuming the mantle of G.C. Williams in this article.3 The reason is because Williams’ theoretical claim has withstood the test of time but his empirical claim has not. Higher-level selection is often a significant and sometimes even a dominating evolutionary force in nature, especially in the case of human cultural evolution. This means that instead of categorically denying the importance of higher-level selection, the balance between levels of selection must be worked out on a case-by-case basis. Williams himself changed his mind for the specific traits of disease virulence, female-biased sex ratios (discussed in Sober and Wilson 1998, p. 35-50) and sexual reproduction (Williams 1971, p. 161; discussed in Distin (2023, p 44).
Another complicating factor was the development of other theoretical frameworks, such as inclusive fitness theory (also called kin selection), selfish gene theory, and evolutionary game theory, which were first regarded as alternatives to multilevel selection but then turned out to be different methods of accounting for the same causal processes (Hamilton 1975; Sober and Wilson 1998; Okasha 2006; Henrich 2004; Wade 2016; summarized in Wilson 2015, ch. 3). Ongoing debates at the expert level are centered largely on the equivalence and relative merits of alternative accounting methods, as described in more detail below.
First, however, it is important to introduce multilevel selection theory on its own terms.
The key to applying Williams’ Rule is to make a nested series of relative fitness comparisons. Are there fitness differences among genes within single organisms? If so, then unless they are highly orchestrated (such as the adaptive component of the immune system), they are likely to result in disruptive traits such as meiotic drive and cancer (Agren 2021; Aktipis 2020; Howe et al 2022). In the case of cancer, cells that proliferate at the expense of neighboring cells have a relative fitness advantage, even if they disrupt the economy of the body and ultimately result in their own destruction with the death of the organism.
Frameshifting upward, are there fitness differences among individuals within single-species social groups? If so, then unless they are highly orchestrated (such as reputational systems that align status with positive contributions to the group), they are likely to result in disruptive traits such as free-riding (compared to providing social benefits), deception (compared to telling the truth), and bullying (compared to peaceful coexistence).
Frameshifting upward yet again, are there fitness differences among groups in a multi-group population? If so, then they may result in impressive cooperation within groups, but also result in highly disruptive behaviors between groups, such as warfare. Cooperation between groups would require a process of selection among groups-of-groups.
Employing this nested series of relative fitness comparisons is not rocket science and in many ways is deeply intuitive for people without specialized training, as I will soon show. Comprehensive treatments are available elsewhere (in addition to the above cited references, see Hertler et al. 2020; Kline et al., 2018; Waring et al. 2015). Since one goal of this article is to provide a tutorial, I will attempt to establish an intuition with a series of examples drawn from both the genetic evolution of nonhuman species and human cultural evolution.
Establishing an Intuition
The game of Monopoly: Imagine playing the game of Monopoly, where the goal is to capture all the real estate and drive the other players bankrupt. This is a process of competition among individuals within a single group, pure and simple. There is almost no context for cooperation among the players, except when those who are losing gang up on those who are winning so that they can ultimately turn on each other.
Now imagine playing in a Monopoly tournament, where the trophy goes to the team that collectively develops its real estate the fastest (e.g., the total number of houses and hotels). Now cooperation becomes the entire context for interactions within groups as they compete with other groups to win the trophy. But there is still no context for cooperation between the teams.
This example shows how intuitive the logic of multilevel selection can be for people without specialized training. It seems that we almost instinctively recognize the context of competition within a single group, compared to competition among groups, and know how to play both games well.
Mean and kind chickens: Imagine that you are a poultry breeder who wants to select a better strain of egg-laying hen.4 The hens are housed in groups. In one experiment you select the most productive hen within each group to breed the next generation of hens. In a second experiment, you select the most productive groups and use all the hens within these groups to breed the next generation of hens.
If you are starting to acquire an intuition about multilevel selection, then you might be able to guess the outcome of the two experiments, which were conducted by the animal breeding scientist William Muir (Muir et al. 2010; discussed in Muir and Wilson 2023). The first experiment was like the single game of Monopoly. The most productive egg layer in each group was the biggest bully, who achieved her productivity by suppressing the productivity of the other hens. Remember—evolution within groups is all about relative fitness. Bullying is a genetically heritable trait in chickens, so five generations of within-group selection resulted in a hyper-aggressive strain of hens who plucked each other’s feathers and murdered each other in their incessant attacks. Egg productivity plummeted, even though the most productive hen within each group was selected generation after generation.
The second experiment was like the Monopoly tournament, selecting for docile traits, so that groupmates didn’t interfere with each other and perhaps even engaged in active teamwork. Egg productivity increased 160% in five generations. A similar experiment was conducted on quail, selecting within and between groups for growth rate, with similar results (Muir et al. 2013).
My chicken example is much like my Monopoly example, but most animal breeders, other than Muir, did not begin with the intuition that between-group selection is far superior to within-group selection for the artificial selection of useful traits in domestic animals. Instead, there was an initial assumption that a trait such as egg productivity or growth rate is a property of individuals and therefore could be selected at the individual level. After all, it is individuals who lay eggs and grow fast or slow. The idea that traits which can be measured in individuals are the product of social interactions, and therefore likely to be disrupted by within-group selection, was counter-intuitive, which made Muir’s experiments an important contribution to the animal breeding literature.
Mean and kind water striders: An example of natural selection, comparable to the artificial selection experiments on chicken and quail, was worked out by my former PhD student, Omar Eldakar (Eldakar et al., 2009a,b, 2010). Water striders are insects that are adapted to skate over the surface of ponds and streams, where they hunt for prey. Males vary in their aggressiveness toward females. In human terms, some can only be described as sexual predators, while others act more like gentlemen. Omar created groups of six males and six females, in which the composition of the males varied from all aggressive, to all passive, and various mixes in between.
Within each group, aggressive males had a decisive mating advantage over the passive males, just like the first chicken experiment. However, groups with mostly passive males were more productive than groups with mostly aggressive males, because the females were not being harassed and could therefore feed and lay eggs.
Because within- and between-group selection were both strong forces, the result was to maintain individual differences among the males. Aggressiveness was being maintained by within-group selection and passiveness was maintained by between-group selection. It is important to appreciate that while adaptive mixtures of behavior are sometimes good for the group, this particular mix is not. Groups would be best off with only passive males and the aggressive males, like the bullying hens, are behaving disruptively for their own benefit. Evolution does not make everything nice!
Since between-group selection requires variation among groups, what causes groups to vary in water strider populations? In one of Omar’s experiments, he allowed both males and females to freely move among groups. Females who entered a group containing aggressive males left as soon as they could. Aggressive males were free to move also, but when all was said and done, there was a significant clustering of females around the passive males. As we shall see, the ability to choose one’s social partners plays an important role in human social interactions.
What are beaver ecosystems good for? Beavers are an amazing example of a keystone species and “ecosystem engineer”, modifying whole landscapes with their dam building activities (Brazier et al.; 2021; Bailey et al., 2004). If we want to understand the nature of beaver ecosystems, the first question to ask is “what’s in it for the beavers?” Flooding the habitat makes clear sense as a defense against terrestrial predators. A preference for trees that are not defended by toxins makes clear sense from the standpoint of their dietary preferences.
Beavers have a major impact on ecosystem properties such as biodiversity and nutrient cycling. Is there any warrant for saying that beaver ecosystems evolved to be diverse or to efficiently cycle nutrients? The alternative hypotheses is that the ecosystem properties are nonadaptive byproducts of beavers that evolved to enhance their own fitness. For example, flooding the habitat makes it less suitable for many species, in addition to making it more suitable for other species. By eating the most palatable trees, beavers leave the trees that are best protected by toxic compounds such as tannins. When these compounds leach into the water, they retard nutrient cycling and degrade the environment for many species of aquatic animals. Even if beaver activity does have a net positive effect on biodiversity and nutrient cycling, this could still be a nonadaptive byproduct of lower-level selection and not an adaptive product of higher-level selection.
Williams argued for conservatism in invoking higher-level selection and I concur. It’s not as if ecosystem-level selection is impossible (see below); only that there is a burden of proof to demonstrate a process of selection at the level of whole beaver ecosystems, which is strong enough to counteract lower-level selection in other directions.
Thanks in part to the influence of Williams, evolutionary ecologists have largely abandoned the simplistic notion that nature, left to itself, strikes some kind of harmonious balance or that ecosystems automatically evolve to be diverse and efficient in their processes (Bodkin 1990, Bodkin and Runte 2016). Instead, ecosystems are envisioned as complex dynamical systems that are often out of equilibrium or can settle into multiple locally stable equilibria. The word “regime” is used to describe a local equilibrium (e.g., Andersen et al., 2009), which aptly invokes what we already know about human political regimes. A political regime has a degree of stability, or else we wouldn’t use the word, but this says nothing about how well the regime functions for the common good. Human regimes can be despotic (organized to benefit the most powerful members of the society) in addition to egalitarian (organized for the common good) and the same is true for biological regimes.
What are phoretic mite communities good for? Having argued for caution in interpreting multispecies ecosystems as adaptive units, I will now show that this interpretation can be fully warranted when selection operates at the appropriate level. Phoresy is the use of one species as a form of transport by another species. For example, most species of dung, carrion, and bark beetles carry a community of mites on their bodies, which disembark and breed alongside their beetle hosts. The mite progeny then climb aboard the beetle progeny to repeat the cycle. A single beetle can carry as many as five species of mites, numbering in the hundreds, tucked into various nooks and crannies of the beetle’s body.
Phoretic associations are interesting because the beetles are a common-pool resource for the mites. Within every community, selection will favor mites that reproduce faster than other mites, even if their activities interfere with the reproduction of the beetle. But mite communities that do not harm and perhaps even enhance the fitness of their beetle hosts will contribute more dispersers than communities that harm their beetle hosts. Unlike beaver ecosystems, with phoretic mite communities there is clearly a process of community-level selection that can oppose the process of within-community selection. The relative strengths of the two levels of selection must be determined empirically.
In my own research on burying beetles (a kind of carrion beetle that specializes on small carcasses) and their phoretic mites, I showed that the mites did not interfere with the reproductive success of their beetle hosts, although they did not have a mutualistic effect either (Wilson 1983). In other studies of beetle-mite associations, the mites have been shown to have a mutualistic effect (reviewed in Wilson 1980; Sun 2022; Seeman and Walter 2023). Hence, phoretic associations provide solid empirical support for selection at the level of multi-species communities.
Community and Ecosystem-level selection in the laboratory: Laboratory experiments similar to the second chicken experiment, where groups of hens were selected for their collective egg-laying productivity, provide more evidence that selection can take place at the level of single-species groups, multi-species communities and ecosystems (reviewed by Wade 2016). I was among the first to conduct ecosystem-level selection experiments with my PhD student William Swenson. In one experiment (Swenson et al. 2000a), we grew plants (Arabidopsis) in sterilized soil to which we added six grams of unsterilized soil from a single well-mixed source. After 35 days, we measured the above-ground biomass of each plant. In a conventional artificial selection experiment, we would have used the seeds from one end of the distribution (i.e., the largest or the smallest plants) to breed the next generation of plants. Instead, we used the soil from underneath the largest (or smallest) plants to breed the next generation of plants, using a common stock of seeds. We were selecting at the level of soil ecosystems. In a second study (Swenson et al. 2000b), we created aquatic microcosms by adding one ml of unsterilized pond water from a single well-mixed source to 28 ml of sterilized liquid medium, incubated the microcosms for four days, and selected them on their ability to degrade a toxic compound (Chloroaniline).
Six grams of soil or one ml of pond water contain millions of microbes comprising dozens or even hundreds of species. Since each unit received its inoculum from a single well-mixed source, initial variation among the units based on sampling error was vanishingly small. Nevertheless, when the units were measured after a period of time (plant biomass, or degradation of the toxic compound), there was plenty of phenotypic variation to select upon. How is that possible? Because each microcosm was a complex system. In complex systems, tiny initial differences among replicate units don’t remain tiny. Instead, they amplify over time. This is called sensitive dependence on initial conditions, which also explains why the weather is so difficult to predict (the so-called “butterfly effect”, described for a general audience by Gleick 1987).
Not only was there variation at the level of ecosystems to select upon, but there was also a response to selection. In other words, the plants became larger in the soil microcosm experiment and the toxic compound became more degraded in the aquatic microcosm experiments. Ecosystem-level selection had demonstrably taken place.
Let’s take stock of our progress so far. One goal of this article is to establish an intuition about Williams’ Rule using biological examples, since it is within biology that Williams’ Rule has become firmly established. Williams’ Rule is not a categorical rejection of higher-level adaptations. Instead, it demands that higher-level adaptations should be invoked only as products of higher-level selection. Every invocation needs to be investigated on a case-by-case basis by making the nested series of relative fitness comparisons.
Establishing an intuition about Williams’ rule is not hard. My Monopoly example provides a form of instant recognition that selection within groups results in ruthlessly competitive behavior, that selection among groups results in teamwork that does not necessarily extend to other groups, and so on. Yet, this recognition is easily obscured by other ways of thinking, which is why it did not occur to animal breeders until Bill Muir brought it to their attention with his experiments.
Keeping Williams’ rule in mind enables us to spot both the presence and absence of functional organization. A single organism is functionally organized, except when impaired by disease or rogue elements such as cancer and meiotic drive. Water striders are adaptive as individuals but not as populations. As populations, they would do much better without the aggressive males. Beavers are adaptive as individuals and small social groups, but beaver ecosystems are probably not adaptive as whole systems. Yet, phoretic communities might well be adaptive as whole systems, thanks to a process of system-level selection. And laboratory experiments provide strong support for the possibility of whole ecosystems as units of selection.
Levels of selection are seldom all or none. Even single multicellular organisms, which are the quintessential example of an adaptive unit, have not entirely suppressed disruptive within group selection such as cancer and meiotic drive genes. Water strider populations reflect both within-group selection (favoring aggressive males) and between-group selection (favoring docile males). Perhaps some aspects of beaver ecosystems have been selected at the level of the whole system. Let’s form some specific hypotheses and test them! Williams’ conservative stance, placing the burden of proof on those who invoke higher-level adaptations to provide evidence for higher-level selection, remains a good research strategy to avoid “just-so” storytelling (Gould and Lewontin 1979).
Williams’ Rule and Human Cultural Evolution
Even though Darwin’s theory of evolution has proven its explanatory scope within the biological sciences, it was confined to the study of genetic evolution for most of the 20th century. Starting in the 1960’s, a few bold thinkers went back to basics and defined evolution the way that Darwin did—as any process combining the triad of variation, selection, and replication, no matter what the underlying mechanisms (e.g., Campbell 1960, 1974; Plotkin 1994). Other mechanisms of inheritance, in addition to genetics, include epigenetics (changes in gene expression rather than gene frequency), forms of social learning found in many species, and forms of symbolic thought that are distinctively human (Jablonka and Lamb 2006).
By now, “generalized Darwinism” is in full swing and extends to all branches of human-related knowledge, which I have called “completing the Darwinian revolution” (Wilson 2019; see also Henrich 2015, 2020; Laland 2017, Hodgson and Thorbjorn 2010; Richerson & Boyd 2005). A recent article details how the new theory leads to practical applications, using the topic areas of complex systems science and engineering, economics and business, mental health and wellbeing, and global change efforts as examples (Wilson et al., 2023).
Continuing the tutorial part of this article, the following sections show that Williams’ Rule is as relevant to human cultural evolution as to biological evolution.
We are a highly group-selected species: The balance between levels of selection is not static but can itself evolve. When between-group selection sufficiently dominates within-group selection, social groups become so cooperative that they invite comparison to single organisms. Indeed, every entity that we call an organism is nothing more or less than a highly integrated social group of lower-level elements that led a more fractious and independent existence in the distant past. As Strassman and Queller (2010, p 605) put it: “What makes an organism is high and near-unanimous cooperation among its constituent parts, with actual conflicts among those parts largely absent or controlled.” This is called a Major Evolutionary Transition (MET) in individuality. Our species is part of the elite club that has undergone a MET, from groups of organisms to groups as organisms (Wilson et al., 2008). Szathmary (2015, p 7) lists some of the features of the human major transition that are shared with other major transitions in the history of life:5 “We see key elements that are highlighted in other transitions: cooperation (including reproductive leveling and food sharing), a form of eusociality, a powerful novel inheritance system, and living in groups.”
The capacity for symbolic thought is a group-level adaptation: One form of cooperation that evolved in our species is the ability to transmit learned information across generations with the help of a shared inventory of symbols. The capacity for cumulative cultural evolution first evolved by genetic evolution and has been co-evolving with it ever since. This is called dual inheritance theory (Richerson and Boyd 2005; Richerson 2017). While the two forms of evolution differ in important details, both take place at multiple levels. In other words, a culturally derived trait can evolve by virtue of benefitting individuals relative to others within the same group, by groups relative to other groups within a multi-group population, and so on for a nested hierarchy of groups. This makes Williams’ Rule as relevant for cultural evolution as for genetic evolution.
Mean and kind Humans: Our behavioral flexibility as individuals enables us to respond adaptively to our immediate environments. This is why we can play the single game of Monopoly and turn on a dime to act as team players in a Monopoly tournament. It follows that whenever human social environments are structured to resemble the first chicken experiment, ruthlessly competitive behavior will be selected, as surely as it evolves by genetic evolution. Thankfully, the same is true for social environments structured to resemble the second chicken experiment.
This is not just idle speculation. The political scientist Elinor Ostrom identified eight core design principles (CDPs) that enable groups to self-manage common-pool resources such as forests, pastures, fisheries and the groundwater. In my collaboration with Ostrom and her associate Michael Cox, we generalized the CDPs to apply to all forms of cooperation (Wilson et al. 2013). In a nutshell, the CDPs suppress the potential for the expression of disruptive self-serving behaviors within groups (like the single game of monopoly and the first chicken experiment) so that succeeding as a group becomes the primary unit of selection (like the monopoly tournament and the second chicken experiment; Wilson et al., 2020). Appreciating the relevance of Williams’ Rule can result in a quantum jump of good governance and good behavior across all scales and contexts (Atkins, Wilson, and Hayes 2018; Wilson et al. 2013).
Literacy and Conflicting Narratives
The worldwide community of people working toward positive change is highly diverse, including formal efforts designated by the word “policy” and informal efforts that individuals and groups engage in all the time. The word “evolution” might be used, but very few change agents have a background in evolutionary biology, much less the developments of the last few decades. It is therefore unsurprising that basic awareness of multilevel selection is low and misunderstandings of adaptation, of the sort that Williams addressed in Adaptation and Natural Selection, are common.
In addition, just as animal breeders didn’t appreciate the relevance of Williams’ Rule because they thought they were selecting individual-level traits rather than social traits, other change agents can be blinded by their narratives. Here are three narratives that interfere with appreciation of Williams’ Rule.
First, the narrative that nature, left to itself, strikes some kind of harmonious balance unless disrupted by human activities (Gowdy et al., 2013). A related narrative is that indigenous societies are inherently cooperative and respectful of their natural resources. This is the naïve “for the good of the group” mentality that motivated Williams to write his book. Against this background, Williams’ Rule helps us to see that in the absence of higher-level selection, natural systems do not necessarily result in what we value, such as the preservation of biodiversity and the provision of ecosystem services. These must be actively selected for. Likewise, all human societies are vulnerable to disruptive lower-level selection and themselves becoming disruptive at higher scales. For example, the first native American tribes to gain access to guns and to master horseback riding used their power advantage against other native American tribes (Gwynne 2011; Silverman 2016). The basic principles of multilevel selection are expected to be culturally universal.
Second, Adam Smith’s metaphor of the invisible hand, which implies that the unregulated pursuit of lower-level interests robustly benefits the common good. This has been the guiding metaphor of neoclassical economics for the last 70 years and Williams’ Rule reveals it to be profoundly false (Wilson and Gowdy 2014). As an historical aside, Smith invoked the metaphor only three times and often appreciated the disruptive effects of lower-level competition in his other writing (Norman 2018, Wright 2005). See Wilson and Snower (2024) for a detailed discussion of multilevel selection as a new paradigm for economics.
Third, complex systems science is oddly tone deaf to Williams’ Rule, which is reflected in the key phrase “complex adaptive system (CAS)”. Consider these examples of CAS listed on Wikipedia: cities, ecosystems, ant colonies, traffic flows, the brain, markets, and the developing embryo.6 Some of these are complex systems that are clearly adaptive as systems because they are units of selection (ant colonies, the brain, and developing embryos). Others are complex systems composed of agents following their own adaptive strategies (cities, ecosystems, traffic flows, and markets), but that doesn’t guarantee that they are adaptive as whole systems. Indeed, the central insight of multilevel selection theory is to point out that unless complex systems are units of selection, they will not be adaptive at the level of the whole system, such as beaver ecosystems compared to phoretic mite communities, to pick two examples provided earlier. As another example, the smart city movement is an effort to make cities function well as whole units, which requires a concerted effort (selecting policies with the welfare of the whole city in mind). In the absence of such an effort, cities do not become smart by themselves.
If complex systems thinkers were attuned to the difference between a complex system that is adaptive as a system (CAS1) and a complex system composed of agents following their respective adaptive strategies (CAS2; Wilson 2016) they would be using the phrase “complex maladaptive system” as often as “complex adaptive system.” Yet, when “complex maladaptive system” was used as a search term, it received zero hits, compared to over 1000 hits for “complex adaptive system” (Wilson and Madhavan 2020). This demonstrates the need for policymakers of all stripes to absorb the insights of generalized Darwinism and multilevel selection theory, in addition to the insights of complex systems theory.
Debates that Continue at the Expert Level
As I mentioned at the beginning of this article, there is a growing community of economists, social scientists, and policymakers who do have a background in evolutionary biology, are aware of Williams’ Rule, and do not need a basic tutorial for themselves (e.g., Henrich 2004; Waring et al. 2015; Brooks et al., 2018; Kline et al., 2018). However, this does not mean that the experts have reached agreement on all issues! Here is a sample of issues that are still being debated.
Equivalence. The underlying unity of all theories of social evolution is still not universally acknowledged among the experts. Wilson and Wilson (2007, p. 345) expressed this unity with the passage “Selfishness beats altruism within groups. Altruistic groups beat selfish groups. Everything else is commentary." Henrich (2004, p. 5) expressed the same unity with the passage “[A]ll solutions to the evolution of altruism—whether they are based on kinship, reciprocity, or group selection…are successful according to the degree in which ‘being an altruist’ predicts that one’s partners or group members are also altruistic“. Yet, major thinkers such as Dawkins (1994) and Pinker (2012) still treat different theoretical frameworks as if they invoke different causal mechanisms, such that one can be rejected in favor of the other (see Figueredo et al. 2020 and Wade 2016 for historical reviews). Authors writing on cultural evolution sometimes maintain that genetic group selection is weak or absent in nature and only strong in the case of human cultural evolution (e.g., Bell et al. 2009; Kline et al. 2018 p. 13).
Here is one way to stress the underlying unity of all theories of social evolution, whether genetic or cultural: Imagine that you are studying the social behavior of a given species or culture. To calculate the fitness of an individual organism, you will need to know the phenotype of the focal individual and the phenotype of the other individuals with whom the focal individual interacts. Those individuals are the salient group as far as the focal individual is concerned. If the focal individual is contributing to the fitness of others within this group or to the group as a whole (including itself), then it is not maximizing its relative fitness within its group. Something more is required to explain the evolution of such a prosocial behavior and that “something” is the existence of many such groups, variation in the frequency of the prosocial behavior among the groups, and the differential contribution of the groups to total evolving population. These are biological facts of life and all theories of social evolution must abide by them. W.D. Hamilton (1975) was able to grasp this point when he viewed his inclusive fitness theory through the lens of the Price equation (recounted by Harman 2010) and general acknowledgement in the academic literature is long overdue.
Going beyond the assumptions of early theoretical models. Initial attempts to model the evolution of altruism, no matter how they were framed (e.g., group selection, kin selection, reciprocity) tended to make a core set of simplifying assumptions. First, altruism was defined as a behavior that decreases the absolute fitness of the actor (-c) and increases the absolute fitness of a single recipient (+b). Second, altruistic and selfish behaviors were assumed to be coded by altruistic and selfish alleles (strict genetic determinism). Given these assumptions, the phenotypic partitioning of variation within and between groups maps directly onto genetic partitioning and above random genetic variation among groups is required for altruism to evolve. The fixation index (Fst) from population genetics, which ranges from zero for random variation among groups to one for complete segregation, is often treated as an index of the relative strength of within- vs. between-group selection (e.g., Bell et al. 2009). As Henrich (2004, p. 16) put it: “Thus, a great deal of theoretical work shows that genetic group selection will only lead to substantial levels of altruism when groups are very small, migration rates are quite low, and the intensity of selection among groups is high compared to the intensity of selection within groups.”
To go beyond these assumptions, let’s take a different starting point: the evolution of a beneficial gene (A, compared to a) in a standard diploid population genetics model. The A gene benefits the whole organism at no relative fitness cost to itself. Within-organism selection is neutral, so any amount of variation among organisms, including random variation, is sufficient for the trait to evolve by between-organism selection. Indeed, the default assumption for diploid population genetics models is random variation (Hardy-Weinberg equilibrium).
Now let’s frameshift upward by considering two types of individual, A and a, interacting in social groups of size N. A-types benefit their whole group at no cost to themselves, compared to freeriding a-types, who accept social benefits without providing them. There are no fitness differences within groups (within-group selection is neutral) so the A trait evolves given any variation between groups, including random variation. To repeat: between-group selection is the only evolutionary force in this model, even when Fst is zero. We can even add a small cost to providing the public good and random variation is still sufficient for the trait to evolve by between-group selection, as long as the net absolute benefit for the A-type is positive. This is altruism defined in terms of relative fitness, as opposed to altruism defined in terms of absolute fitness (Wilson 1990).
In short, there is a whole class of no-cost and low-cost public good provisioning behaviors that do not require high Fst values to evolve by between-group selection. An example is group-level decision-making, where pooling and integrating information is not individually costly and can result in large collective benefits. Thus, collective intelligence is not restricted to groups with high genetic relatedness such as the eusocial insects, where it has been well-documented, but also has evolved in social groups such as African buffalo herds, where genetic relatedness is much lower (Prins 1995; discussed in Wilson 1997, p 132).
Things get even more interesting when we complicate the genotype-phenotype relationship (reviewed by Wade 2016). Whenever single-species groups or multi-species communities are formed in the laboratory, there is more phenotypic variation among the units than expected on the basis of a one-to-one mapping of genes onto behaviors (Goodnight and Stevens 1997). Instead, genes code for component traits that interact with each other to produce the phenotypic trait that is being selected. When the unit of selection is a complex system, small differences between units don’t remain small but tend to magnify over time—sensitive dependence on initial conditions. This means that phenotypic variation among units will almost never be in short supply—in contrast to the core assumptions of the early models, which predict that phenotypic variation will decline with initial group size and horizontal mixing among groups. Decades of research by Michael Wade and his associates (recounted in Wade 2016) and my ecosystem selection experiments with Bill Swenson demonstrate the power of complex interactions to generate variation among groups, even when initial variation due to sampling error is vanishingly small.
Just because there is phenotypic variation among units doesn’t mean that it is heritable. In artificial selection experiments at the individual level, a response to selection is proof of heritability (Falconer 1981). For example, if you select for a measurable trait in Drosophila, such eye color, and the frequency of the trait increases in the offspring generation, then the trait was heritable. By the same token, a response to selection in a group-level or ecosystem-level selection experiment is proof of heritable variation at these levels, no matter how complex and poorly understood the proximate causal mechanisms (Goodnight 2000, Wade 2016). The academic literature on these issues is still highly unsettled. For example, some authors argue that microbiomes can’t be units of selection because there is too much horizontal mixing and not enough vertical transmission (e.g., Doolittle and Inkpen 2018; Haag et al. 2018; Lean et al. 2022; Roughgarden 2022). This reasoning is based on the early simplifying assumptions outlined above and does not reflect more recent thinking based on complex interactions. Ultimately, determining the heritability of variation in microbiomes is an empirical matter.
In his (for the most part) excellent theoretical overview, Henrich (2004; p. 16-17) draws upon complex systems theory to emphasize the importance of multiple basins of attraction. In the standard altruism-selfishness model, the altruistic trait declines in frequency within each group. In contrast, multiple basins of attraction are locally stable by definition. If some local equilibria function better at the group level than others, then selection can take place among equilibria and the final outcome can be favored by both within- and between-group selection (Boyd and Richerson 1990). For Henrich, this is one reason why group selection is a strong force in human cultural evolution. But multiple basins of attraction also exist throughout the biological world, including the concept of ecological regimes discussed earlier. For both natural and human social systems, we must go beyond the limited assumptions of the early core models to include the much richer field of possibilities afforded by a combination of complex systems theory and multilevel selection theory.
Using Williams’ Rule to Inform Positive Change Efforts
I hope that I have succeeded in showing the relevance of Williams’ Rule for identifying both the presence and absence of functional organization for any process that combines the ingredients of variation, selection, and replication (generalized Darwinism). This makes it an immensely practical tool for positive change efforts of all sorts. The reason that biological and human social systems often fail to behave adaptively is due to agents within the system pursuing their own adaptive strategies, to the short-term detriment of other agents and the long-term detriment of the system as a whole. In these cases, selection at the scale of the whole system is required for it to become adaptive, which often involves actively suppressing lower-level selection pressures. This is the distinction between two meanings of complex adaptive systems described earlier.
Sometimes, groups achieve a high level of cooperation on their own, without requiring the assistance of conscious change agents. For example, some of the common-pool resource groups studied by Elinor Ostrom (1990; see Cox et al. 2010 for an additional sample of groups) had implemented the core design principles without needing to be coached, which is how Ostrom was able to derive them in the first place. The flipside of this coin is that many other common-pool resource groups did not implement the core design principles on their own and therefore were not sustainably managing their resources. Work is required for these groups to become more sustainable, which often involves addressing structural imbalances in addition to educative efforts.
The generality of Williams’ Rule helps us to see that what Ostrom showed for common-pool resource groups applies to all cooperative endeavors (Wilson et al. 2013; Wilson et al. 2020). No matter what the specific context (e.g., business, agriculture, education, biodiversity), some groups will have achieved a high degree of cooperation on their own but many others will require some sort of assistance. And even the high-performing groups, despite being internally sustainable, might be undermining social welfare at larger scales.
My hope for this largely tutorial article is to equip the reader with an intuition about multilevel selection, as preparation for reading the rapidly expanding literature where more in-depth treatments and applications are available (e.g., Wilson et al. (2023) for policy applications in general; Wilson and Snower (2024) for economics; Hayes et al. (2020) for clinical psychology; and a recent special issue devoted to evolution and sustainability (Jorgenson et al. 2023). The basic intuition that flows from MLS theory includes the following major points.
• The welfare of the whole earth system must be the ultimate unit of selection. Any other target of selection runs the risk of becoming disruptive at higher scales. Superficially, it might seem that selection at the planetary scale is impossible because our planet is not competing with any other planets. What makes planet-level selection possible is a decision-making process that makes planetary welfare the target of selection, orients variation around the target, and identifies and replicates better practices, realizing that they will be sensitive to context. This is how conscious cultural evolution takes often takes place at smaller scales, as opposed to a raw process of selection among multiple groups, and can also take place at the global scale.
• Small, purpose-oriented, and appropriately structured groups remain a fundamental unit of human society and can be regarded as the “cells” of larger-scale social organizations (Wilson and Coan 2021). This point was almost entirely eclipsed by reductionistic thinking for nearly a century, including the rational actor model in economics, methodological individualism in the social sciences, and selfish gene thinking in my field of evolutionary biology. Anyone can start working at the scale of small groups—their families, neighborhoods, schools, businesses, churches, and volunteer activities, to improve their wellbeing as individuals and the efficacy of their actions.
• Between the global scale and small groups, there is a veritable tropical rain forest of meso-scale groupings and social identities, such as a person’s nationality, ethnicity, gender, religion, political affiliation, and the multilevel institutional structure of any large-scale society. These identities are part of our cultural evolutionary past and the starting point for future cultural evolution. We cannot make them go away, and more than we can make our personal histories go away. In many cases they deserve to remain strong--as long as they are coordinated with the global good in mind.
Conclusions
The implication of Williams Rule for positive change efforts is that a consciously constructed cultural inheritance system is required. A sustainable system must be the explicit target of selection, appropriate variation must be oriented around the target, better practices must be identified and replicated (realizing that they are likely to be sensitive to context) and the process must be reiterated again and again in continuous improvement cycles.
That’s a tall order, especially when the target of selection is a coupled human and natural system at the scale of the whole earth. But no amount of effort will succeed without an appreciation of Williams’ Rule. I hope that this article will begin to accomplish for all positive change agents what Williams accomplished for evolutionary biologists with his book Adaptation and Natural Selection.
Acknowledgements
I thank Tim Waring, Joan Roughgarden, and the anonymous reviewers for their constructive comments. Supported by a grant from the John Templeton Foundation (#62318).
Notes
1. Williams was not alone, of course. Other biologists who shared his views included David Lack (1954) and John Maynard Smith (1964; discussed by Gardner 2013, 2014). Also, some biologists such as V.C. Wynne-Edwards (1962) did appreciate that group-level selection is required for the evolution of group-level adaptations, but regarded group-level selection as far more pervasive than warranted by the evidence. Williams critiqued both of these positions. See Borrello (2010) for a book-length history of the group selection controversy.
2. My use of the term “disruptive” refers to traits that are favored by within-group selection, which interfere with group-level functional organization. This usage is not to be confused with the term “disruptive selection” in the population genetics literature, which refers to selection for the extremes of a normal phenotypic distribution, in contract to normalizing selection, which selects for the mean.
3. Another early proponent of group selection was Michael Wade, who summarizes the research of himself and his associates in Wade (2016).
4. This example is featured in a National Geographic podcast titled “The Problem with Superchickens”: https://www.nationalgeographic.com/podcasts/article/episode-31-the-problem-with-superchickens
5. The human MET also differs from other METs in some respects, as discussed by Bourke (2022), Patten et al. (2023), and West et al. (2015).
6. https://en.wikipedia.org/wiki/Complex_adaptive_system
References
Agren, A. (2021). The Gene’s-Eye View of Evolution. Oxford University Press.
Aktipis, A. (2020). The Cheating Cell: How Evolution Helps Us Understand and Treat Cancer. Princeton University Press.
Andersen, T., Carstensen, J., Hernández-García, E., & Duarte, C. M. (2009). Ecological thresholds and regime shifts: Approaches to identification. Trends in Ecology & Evolution, 24(1), 49–57. https://doi.org/10.1016/j.tree.2008.07.014
Atkins, P. W. D., Wilson, D. S., & Hayes, S. C. (2019). Prosocial: Using evolutionary science to build productive, equitable, and collaborative groups. New Harbinger.
Bailey, J. K., Schweitzer, J. A., Rehill, B. J., Lindroth, R. L., Martinsen, G. D., & Whitham, T. G. (2004). Beavers as molecular geneticsts: A genetic basis to the foraging of an ecosystem engineer. Ecology, 85, 603–608. https://doi.org/10.1890/03-3049
Bell, A. V., Richerson, P. J., & McElreath, R. (2009). Culture rather than genes provides greater scope for the evolution of large-scale human prosociality. Proceedings of the National Academy of Sciences, 106(42), 17671–17674. https://doi.org/10.1073/pnas.0903232106
Bodkin, B. B. (1990). Discordant Harmonies: A new ecology for the twenty-first century. USA, Oxford University Press USA.
Botkin, D. B., & Runte, A. (2016). 25 Myths That Are Destroying the Environment: What Many Environmentalists Believe and Why They Are Wrong (Illustrated edition). Taylor Trade Publishing.
Borrello, M. (2010). Evolutionary Restraints: The Contentious History of Group Selection. University of Chicago Press.
Bourke, A. F. G. (2011). Principles of Social Evolution (p. 280). OUP Oxford. http://www.amazon.co.uk/Principles-Social-Evolution-Oxford-Ecology/dp/0199231168
Boyd, R., & Richerson, P. J. (1990). Group selection among alternative evolutionarily stable strategies. Journal of Theoretical Biology, 145(3), 331–342. https://doi.org/10.1016/S0022-5193(05)80113-4
Brazier, R. E., Puttock, A., Graham, H. A., Auster, R. E., Davies, K. H., & Brown, C. M. L. (2021). Beaver: Nature’s ecosystem engineers. WIREs Water, 8(1), e1494. https://doi.org/10.1002/wat2.1494
Brooks, J. S., Waring, T. M., Borgerhoff Mulder, M., & Richerson, P. J. (2018). Applying cultural evolution to sustainability challenges: An introduction to the special issue. Sustainability Science, 13(1), 1–8. https://doi.org/10.1007/s11625-017-0516-3
Campbell, T. D. (1960). Blind variation and selective retention in creative thought and other knowledge processes. Psychological Review, 67, 380–400.
Campbell, D. T. (1974). Evolutionary epistemology. In P. A. Schilpp (Ed.), The Philosophy of Karl Popper (pp. 413–463). Open court publishing.
Cox, M., Arnold, G., & Villamayor-Tomas, S. (2010). A review of design principles for community-based natural resource management. Ecology and Society, 15. http://www.ecologyandsociety.org/vol15/iss4/art38/
Dawkins, R. (1994). Bury the vehicle. Behavioral and Brain Sciences, 17, 616–617.
Distin, M. R. (2023). Evolution in Space and Time: The Second Synthesis between Ecology, Evolutionary Biology, and the Philosophy of Biology. https://hdl.handle.net/10550/85722
Doolittle, W. F., & Inkpen, S. A. (2018). Processes and patterns of interaction as units of selection: An introduction to ITSNTS thinking. Proceedings of the National Academy of Sciences, 115(16), 4006–4014. https://doi.org/10.1073/pnas.1722232115
Eldakar, O. T., Dlugos, M. J., Pepper, J. W., & Wilson, D. S. (2009a). Population structure mediates sexual conflict in water striders. Science (New York, N.Y.), 326(5954), 816–816.
Eldakar, O. T. D., Michael J. Holt, Galen P. Wilson, David Sloan Pepper, John W. (2009b). Population structure influences sexual conflict in wild populations of water striders. Science, 326(null), 816–816. https://doi.org/10.1126/science.1180183
Eldakar, O. T., Wilson, D. S., Dlugos, M. J., & Pepper, J. W. (2010). The role of multilevel selection in the evolution of sexual conflict in the water strider aquarius remigis. Evolution; International Journal of Organic Evolution, 64(11), 3183–3189.
Falconer, D. S. (1981). Introduction to Quantitative Genetics (2nd ed.). Longman.
Figueredo, A. J., Hertler, S. C., & Peñaherrera-Aguirre, M. (2020). An Intellectual History of Multilevel Selection from Darwin to Dawkins. In S. C. Hertler, A. J. Figueredo, & M. Peñaherrera-Aguirre (Eds.), Multilevel Selection: Theoretical Foundations, Historical Examples, and Empirical Evidence (pp. 1–40). Springer International Publishing. https://doi.org/10.1007/978-3-030-49520-6_1
Gardner A (2013) Adaptation of individuals and groups. In: From groups to individuals (F Bouchard & P Huneman, eds), MIT Press. P 99-116.
Gardner, A. (2014). Genomic imprinting and the units of adaptation. Heredity, 113(2), Article 2. https://doi.org/10.1038/hdy.2013.128
Gleick, J. (1987). Chaos: Making a new science. Penguin Books.
Goodnight, C. J. (2000). Heritability at the ecosystem level. Proceedings of the National Academy of Sciences USA, 97, 9365–9366.
Goodnight, C. J., & Stevens, L. (1997). Experimental studies of group selection: What do they tell us about group selection in nature? American Naturalist, 150, S59–S79.
Gould, S. J., & Lewontin, R. C. (1979). The spandrels of San Marco and the panglossian paradigm: A critique of the adaptationist program. Proceedings of the Royal Society of London, B205, 581–598.
Gowdy, J., Dollimore, D., Witt, U., & Wilson, D. S. (2013). Economic Cosmology and the Evolutionary Challenge. Journal of Economic Behavior & Organization, in press.
Gwynne, S. C. (2011). Empire of the Summer Moon: Quanah Parker and the Rise and Fall of the Comanches, the Most Powerful Indian Tribe in American History. Scribner.
Haag, K. L. (2018). Holobionts and their hologenomes: Evolution with mixed modes of inheritance. Genetics and Molecular Biology, 41(1 suppl 1), 189–197. https://doi.org/10.1590/1678-4685-gmb-2017-0070
Hamilton, W. D. (1975). Innate social aptitudes in man, an approach from evolutionary genetics. In R. Fox (Ed.), Biosocial anthropology (pp. 133–155). Malaby Press.
Harman, O. (2010). The Price of Altruism: George Price and the Search for the Origins of Kindness. Norton.
Hayes, S. C., Hofmann, S. G., & Wilson, D. S. (2020). Clinical psychology is an applied evolutionary science. Clinical Psychology Review, 81, 101892. https://doi.org/10.1016/j.cpr.2020.101892
Henrich, J. (2004). Cultural group selection, coevolutionary processes and large-scale cooperation. Journal of Economic Behavior and Organization, 53, 3–35.
Henrich, J. (2015). The Secret of Our Success: How culture is driving human evolution, domesticating our species, and making us smarter. Princeton University Press.
Henrich. (2020). The WEIRDEST People in the World: How the West Became Psychologically Peculiar and Particularly Prosperous. Farrar, Straus & Giroux
Hertler, S. C., Figueredo, A. J., & Peñaherrera-Aguirre, M. (2020). Multilevel Selection: Theoretical Foundations, Historical Examples, and Empirical Evidence (1st ed. 2020 edition). Palgrave Macmillan.
Hodgson, G. M., & Thorbjorn, K. (2010). Darwin’s Conjecture: The Search of General Principles of Social and Economic Evolution. University of Chicago Press.
Howe, J., Rink, J. C., Wang, B., & Griffin, A. S. (2022). Multicellularity in animals: The potential for within-organism conflict. Proceedings of the National Academy of Sciences, 119(32), e2120457119. https://doi.org/10.1073/pnas.2120457119
Jablonka, E., & Lamb, M. J. (2006). Evolution in Four Dimension: Genetic, Epigenetic, Behavioral, and Symbolic Variation in the History of Life. MIT Press.
Jørgensen, P., Weinberger, V. P., & Waring, T. M. (2023). Evolution and sustainability: Gathering the strands for an Anthropocene synthesis. Philosophical Transactions of the Royal Society B: Biological Sciences, 379(1893), 20220251. https://doi.org/10.1098/rstb.2022.0251
Kline, M. A., Waring, T. M., & Salerno, J. (2018). Designing cultural multilevel selection research for sustainability science. Sustainability Science, 13(1), 9–19. https://doi.org/10.1007/s11625-017-0509-2
Laland, K. N. (2017). Darwin’s unfinished symphony: How culture made the human mind (p. 450). Princeton.
Lack, D. (1954) The Natural Regulation of Animal Numbers. Clarendon Press, Oxford.
Lean, C. H., Doolittle, W. F., & Bielawski, J. P. (2022). Community-level evolutionary processes: Linking community genetics with replicator-interactor theory. Proceedings of the National Academy of Sciences, 119(46), e2202538119. https://doi.org/10.1073/pnas.2202538119
Maynard Smith, J. (1964). Group selection and kin selection. Nature, 201, 1145–1146.
Muir, William. M., Bijma, P., & Schinckel, A. (2013). Multilevel Selection With Kin And Non‐Kin Groups, Experimental Results With Japanese Quail (Coturnix Japonica). Evolution, 67(6), 1598–1606. https://doi.org/10.1111/evo.12062
Muir, W. M., Wade, M. J., Bijma, P., & Ester, E. D. (2010). Group selection and social evolution in domesticated chickens. Evolutionary Applications, 3, 453–465.
Muir, W. M., Wilson, D.S. (2023). When the Strong Outbreed the Weak: An Interview with William Muir. This View of Life. https://www.prosocial.world/posts/when-the-strong-outbreed-the-weak-an-interview-with-william-muir
Norman, J. (2018). Adam Smith: Father of Economics (Illustrated edition). Basic Books.
Ostrom, E. (1990). Governing the commons: The Evolution of institutions for collective Action. Cambridge University Press.
Okasha, S. (2006). Evolution and the levels of selection. Oxford University Press.
Patten, M. M., Schenkel, M. A., & Ågren, J. A. (2023). Adaptation in the face of internal conflict: The paradox of the organism revisited. Biological Reviews, 98(5), 1796–1811. https://doi.org/10.1111/brv.12983
Pinker, S. (2012). The false allure of group selection. Edge.Org. http://edge.org/conversation/the-false-allure-of-group-selection
Plotkin, H. (1994). Darwin Machines and the nature of knowledge. Harvard University Press.
Prins, H. H. T. (1995). Ecology and Behaviour of the Africa Buffalo: Social inequality and and Decision Making. Springer.
Richerson, P.J., (2017). Recent Critiques of Dual Inheritance Theory. Evolutionary Studies in Imaginative Culture, 1(1), 203–203. https://doi.org/10.26613/esic.1.1.27
Richerson, P. J., & Boyd, R. (2005). Not by genes alone: How culture transformed human evolution. University of Chicago Press.
Roughgarden, J. (2022). Holobiont Evolution: Population Theory for the Hologenome. The American Naturalist, 723782. https://doi.org/10.1086/723782
Seeman, O. D., & Walter, D. E. (2023). Phoresy and Mites: More Than Just a Free Ride. Annual Review of Entomology, 68(1), 69–88. https://doi.org/10.1146/annurev-ento-120220-013329
Silverman, D. J. (2016). Thundersticks: Firearms and the Violent Transformation of Native America (Illustrated edition). Belknap Press: An Imprint of Harvard University Press.
Sober, E., & Wilson, D. S. (1998). Unto Others: The Evolution and Psychology of Unselfish Behavior. Harvard University Press.
Sober, E., & Wilson, D. S. (2011). Adaptation and Natural Selection revisited. Journal of Evolutionary Biology, 24(2), 462–468. https://doi.org/10.1111/j.1420-9101.2010.02162.x
Strassmann, J. E., & Queller, D. C. (2010). The Social Organism: Congresses, Parties, And Committees. Evolution, 64(3), 605–616. https://doi.org/10.1111/j.1558-5646.2009.00929.x
Sun, S.-J. (2022). A framework for using phoresy to assess ecological transition into parasitism and mutualism. Symbiosis, 86(2), 133–138. https://doi.org/10.1007/s13199-022-00830-7
Swenson, W., Wilson, D. S., & Elias, R. (2000). Artificial Ecosystem Selection. Proceedings of the National Academy of Sciences, 97, 9110–9114.
Swenson, W., Arendt, J., & Wilson, D. S. (2000). Artificial selection of microbial ecosystems for 3-chloroaniline biodegradation. Environmental Microbiology, 2, 564–571.
Szathmáry, E. (2015). Toward major evolutionary transitions theory 2.0. Proceedings of the National Academy of Sciences, 112(33), 10104–10111. https://doi.org/10.1073/pnas.1421398112
Wade, M. J. (2016). Adaptation in Metapopulations: How Interaction Changes Evolution. In Adaptation in Metapopulations. University of Chicago Press. https://doi.org/10.7208/9780226129877
Waring, T., Kline, M., Brooks, J., Goff, S., Gowdy, J., Janssen, M., Smaldino, P., & Jacquet, J. (2015). A multilevel evolutionary framework for sustainability analysis. Ecology and Society, 20(2). https://doi.org/10.5751/ES-07634-200234
West, S. A., Fisher, R. M., Gardner, A., & Kiers, E. T. (2015). Major evolutionary transitions in individuality. Proceedings of the National Academy of Sciences, 112(33), 10112–10119. https://doi.org/10.1073/pnas.1421402112
Williams, G. C. (1966). Adaptation and Natural Selection: A critique of some current evolutionary thought. Princeton University Press.
Williams, G. C. (1971). Group Selection (1st edition). Routledge.
Wilson, D. S. (1975). A theory of group selection. Proceedings of the National Academy of Sciences, 72, 143–146.
Wilson, D. S. (1980). The Natural Selection of Populations and Communities (p. 186 pp). Benjamin/Cummings.
Wilson, D. S. (1983). The effect of population structure on the evolution of mutualism: A field test involving burying beetles and their phoretic mites. American Naturalist, 121, 851–870.
Wilson, D. S. (1990). Weak altruism, strong group selection. Oikos, 59, 135–140.
Wilson, D. S. (1997). Altruism and organism: Disentangling the themes of multilevel selection theory. American Naturalist, 150, S122–S134.
Wilson, D. S. (2015). Does Altruism Exist? Yale University Press.
Wilson, D. S. (2016). Two meanings of complex adaptive systems. In Complexity and Evolution: A New Synthesis for Economics (pp. 31–46). MIT Press.
Wilson, D. S. (2019). This View of Life: Completing the Darwinian Revolution. Pantheon/Random House.
Wilson, D. S., & Coan, J. A. (2021). Groups as Organisms: Implications for Therapy and Training. Clinical Psychology Review, 85, 101987–101987.
Wilson, D. S., & Gowdy, J. M. (2014). Human ultrasociality and the invisible hand: Foundational developments in evolutionary science alter a foundational concept in economics. Journal of Bioeconomics, 17(1), 37–52. https://doi.org/10.1007/s10818-014-9192-x
Wilson, D. S., & Madhavan, G. (2020). Complex Maladaptive Systems. The Bridge, 50(4). https://www.nae.edu/244709/Complex-Maladaptive-Systems
Wilson, D.S., Madhavan, G., Gelfand, M., Hayes, S.C., Atkins, P.W.B., Colwell, R. R. (2023). Multilevel Cultural Evolution: From New Theory to Practical Applications. Proc. Nat. Acad. Sci., in press.
Wilson, D. S., Ostrom, E., & Cox, M. E. (2013). Generalizing the core design principles for the efficacy of groups. Journal of Economic Behavior & Organization, 90, S21–S32. https://doi.org/10.1016/j.jebo.2012.12.010
Wilson, D. S., Philip, M. M., MacDonald, I. F., Atkins, P. W. B., & Kniffin, K. M. (2020). Core design principles for nurturing organization-level selection. Scientific Reports, 10(1), 13989–13989. https://doi.org/10.1038/s41598-020-70632-8
Wilson, D.S. & Snower, D.J. (2023). Rethinking the Theoretical Foundation of Economics III: Putting the Multilevel Paradigm to Work. Economics as an Evolutionary Science.
Wilson, D. S., Van Vugt, M., & O’Gorman, R. (2008). Multilevel Selection Theory and Major Evolutionary Transitions: Implications for Psychological Science. Current Directions in Psychological Science, 17(1), 6–9. https://doi.org/10.1111/j.1467-8721.2008.00538.x
Wilson, D. S., & Wilson, E. O. (2007). Rethinking the theoretical foundation of sociobiology. Quarterly Review of Biology, 82, 327–348.
Wright, J. B. (2005). Adam Smith and Greed. Journal of Private Enterprise, 21, 46–58.
Wynne-Edwards, V. C. (1962). Animal disperson in relation to social behavior. Oliver & Boyd.
Header Image by Louis Maniquet on Unsplash